Understanding Black Holes
Black holes are one of the universe‘s most mysterious and compelling phenomena, marrying the strange worlds of quantum mechanics and general relativity.
They challenge our perceptions of space, time, and the very fabric of reality.
Conceptual Foundations
Black holes are regions in space where gravity is so strong that nothing, not even light, can escape their grasp.
This extreme gravity is the result of matter being compressed into a very small area.
The concept of black holes emerges from the general theory of relativity, proposed by Einstein in the early 20th century, which revolutionized our understanding of gravity as a curvature of spacetime caused by mass.
-
Event Horizon: The boundary around a black hole is known as the event horizon.
This is the ‘point of no return,’ where the gravitational pull becomes strong enough that it would require a speed greater than that of light to escape, making it effectively invisible.
-
Singularity: At the core of a black hole lies the singularity, a point of infinite density where the laws of physics as we know them cease to function.
Mass, Space, and Gravity
The mass of a black hole is concentrated at a single point of infinite density, known as the singularity.
It is this mass that warps the surrounding spacetime so dramatically that not even light can escape from the event horizon, creating what we perceive as a black hole.
-
Spacetime: The mass of a black hole significantly affects the spacetime around it.
Near a black hole, spacetime curves so much that the paths taken by particles and light are bent inward towards the event horizon.
-
Gravitational Influence: Despite their often massive size, black holes can be quite small in volume.
This compact mass produces immense gravitational influence that can affect other objects over vast distances, playing a crucial role in the dynamics of the universe, including star formation and galaxy evolution.
Understanding these foundations is essential for grasping the complexities and significance of black holes within our universe.
However, black holes are not just theoretical constructs; they are real objects that have been observed and studied, shedding light on some of the most fundamental questions about our universe’s structure and origins.
Scientific Advancements in Black Hole Research
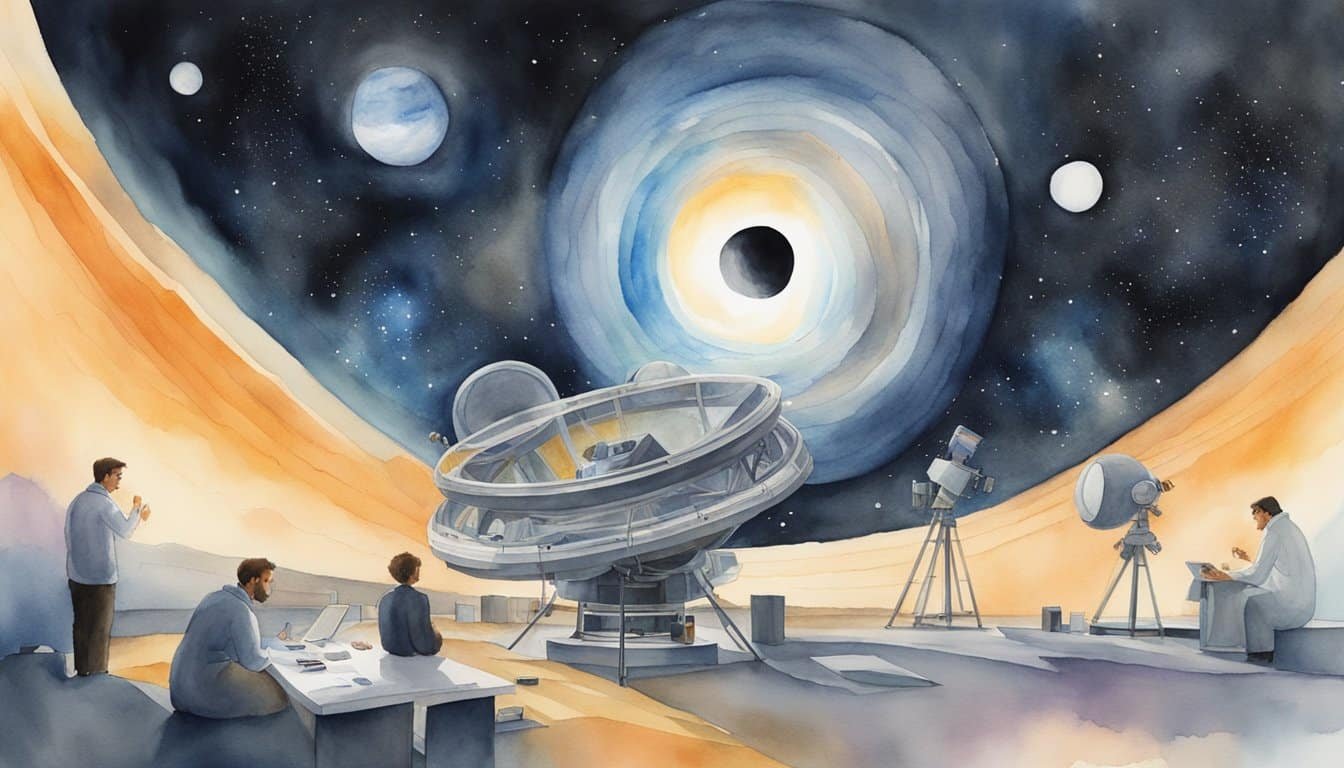
The quest to understand black holes has led to groundbreaking experiments right here on Earth.
Laboratories worldwide are bustling as physicists employ cutting-edge theories and technology to unlock the mysteries of these enigmatic celestial objects.
Experiments Mimicking Black Holes
Laboratories have not quite created real black holes but have achieved the next best thing: simulated versions that exhibit similar characteristics.
For instance, physicist Jeff Steinhauer has made strides in replicating a lab-grown black hole at the Technion-Israel Institute of Technology.
Using a flow of ultra-cold rubidium atoms, his experiment models the event horizon from which, according to Stephen Hawking’s renowned theory, Hawking radiation should emerge.
The groundbreaking finding, which supports Hawking’s predictions, was published in the journal Physical Review Letters, and posits that these lab-created analogues emit what could be termed as the equivalent of this radiation.
The Role of Quantum Mechanics
In these sophisticated experimental set-ups, quantum mechanics play a pivotal role, offering insights into the behavior of particles at the edge of these simulated black holes.
Quantum mechanics provide the framework for understanding how, theoretically, photons and other particles can escape the immense gravity of a black hole, leading to the emission of Hawking radiation.
Through the lens of quantum gravity, researchers like Lotte Mertens delve into the realm of virtual particles and their behavior under such extreme conditions.
This exploration enhances our understanding of both the theoretical underpinnings and the practical representations of black holes, expanding knowledge that could one day answer some of the deepest questions of the cosmos.
Practical Implications and Theoretical Developments
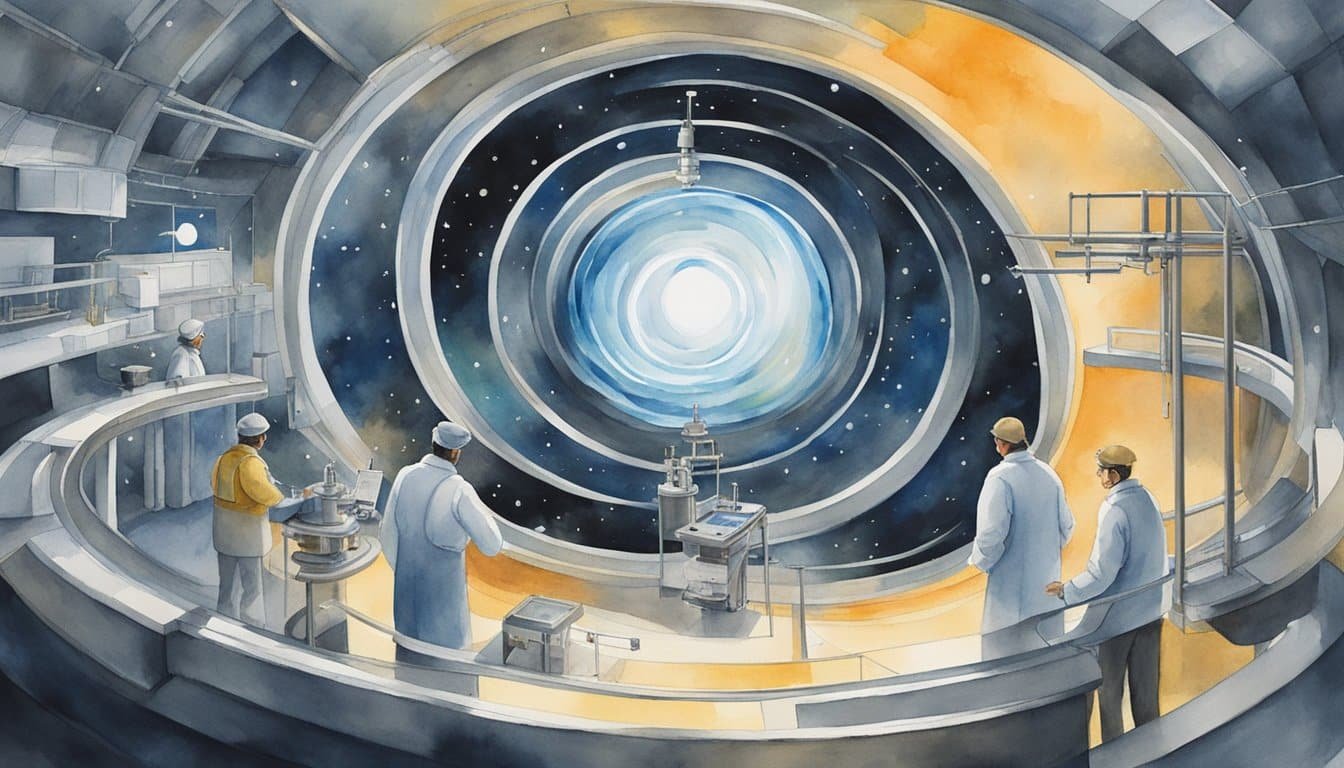
In the realm of astrophysics, creating a black hole in a laboratory setting has far-reaching implications, truly pushing the boundaries of what was once thought to be purely theoretical.
This leap from paper to practice marks a significant milestone for both knowledge acquisition and technological advancement.
Impacts on Astrophysics
The successful creation of black holes in laboratory environments can lead to major breakthroughs in understanding stellar astrophysics.
By studying these small-scale black holes, scientists can observe processes in real-time, such as accretion disk formation and the impacts of radiation on nearby atoms.
For instance, laboratories like the University of Amsterdam might be able to simulate how energy is transferred in these violent cosmic neighborhoods, shedding light on how stars evolve.
Moreover, the ripple effects of such studies stretch into the heart of quantum mechanics.
Theoretical physicists have speculated about the existence of microscopic wormholes and how quantum fluctuations at the event horizon could lead to the discovery of new particles or exotic states of matter, like the Bose-Einstein condensate.
These experiments have tremendous value, providing astronomers with a better understanding of thermal radiation and the dynamic behaviors of stars near black holes.
Future Research and Technology
The technology developed to create black holes could be a precursor to revolutionary tools.
Quantum computers, for example, stand to benefit from this research through a deeper understanding of quantum states influenced by extreme gravity.
This knowledge might one day contribute to building more sophisticated quantum computers, which could have been theoretically discussed at institutions like Princeton.
Additionally, the study of laboratory-created black holes offers a glimpse into the practical applications of harnessing such immense energy.
While the full extent of these applications is still unfolding, the advancements could steer the direction of future energy sources, potentially informing how we tackle the energy challenges on a broader scale.
These developments are not just exciting from a scientific perspective; they carry the promise of transforming our day-to-day technology in ways that are currently difficult to imagine.